ATP synthase, also known as FOF1-ATPase or the F-ATPase, is an evolutionarily conserved enzyme central to the bioenergetics of most living cells. Due to its complex structure and multiple possible mechanisms of inhibition, it is considered an interesting target for new therapeutics. The main biological role of the F-ATPase is to couple the electrochemical proton gradient across the phospholipid bilayer with the synthesis of ATP – the universal carrier of biologically useful energy – from ADP and inorganic phosphate.
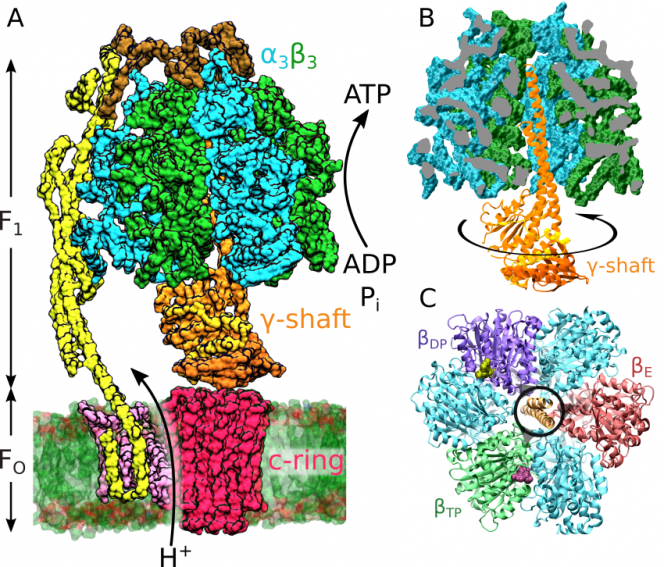
Hence, the F-ATPase is not just a catalyst, but also a nanomachine that can utilize the energy stored in ionic gradient to perform work associated with ATP synthesis under conditions that strongly favor the reverse reaction, i.e. hydrolysis to substrates.
Independently of the particular role played by the F-ATPase in the living world, its mechanism of action is also remarkably unique. The enzyme has been shown to consist of two rotating motor proteins: the bilayer-embedded FO, responsible for the ionic transfer, and the soluble F1, which performs the actual synthesis or hydrolysis reaction, depending on the conditions. In the synthesis mode, each ion transported via FO triggers an abrupt rotation of its rotor part, the so-called c-ring, by one of the 8-15 c subunits. This movement generates an effective torque, enforcing the rotation of the asymmetric γ-shaft buried within the immobile enzyme head composed of hexagonally aligned alternating α and β subunits. The movement of γ in discrete steps results in a sequence of conformational changes within the catalytic sites located in the β subunits. By periodically cycling between conformations with different affinities for substrates and products, the enzyme speeds up the synthesis of ATP, strongly shifting the chemical equilibrium towards the desired product.
Despite many efforts, no consistent microscopic description of the mechanochemical coupling within F-ATPase has been proposed that would explain the observed rotation pattern of γ and its relationship with the sequence of conformational changes within catalytic subunits, as well as the almost ideal thermodynamical efficiency of energy conversion.
By accounting for the thermally activated conformational dynamics of the enzyme, computer simulations allowed for the extension of early semi-quantitative models of the catalytic cycle of ATP synthase, providing a molecular interpretation of single-molecule experiments and elucidating certain aspects of the enzyme's functioning. In particular, our simulations shed light on the distribution of torsional elasticity within F1, believed to be a key factor in the mechanical coupling of both motors. Moreover, we proposed a detailed sequence of conformational changes and a mechanism of energy conversion during the major rotary substep, as well as characterized the structure of F1 trapped in the metastable pre-ATP release state. Our current research aims to obtain a full free energy profile for the rotation-coupled catalysis that would allow for quantitative understanding of the enzyme's intricate mechanism of action, enabling us to rationally design novel selective inhibitors with antimicrobial activity.
Click to play: